Direct Air Capture
Type
Areas of deployment
Proposal
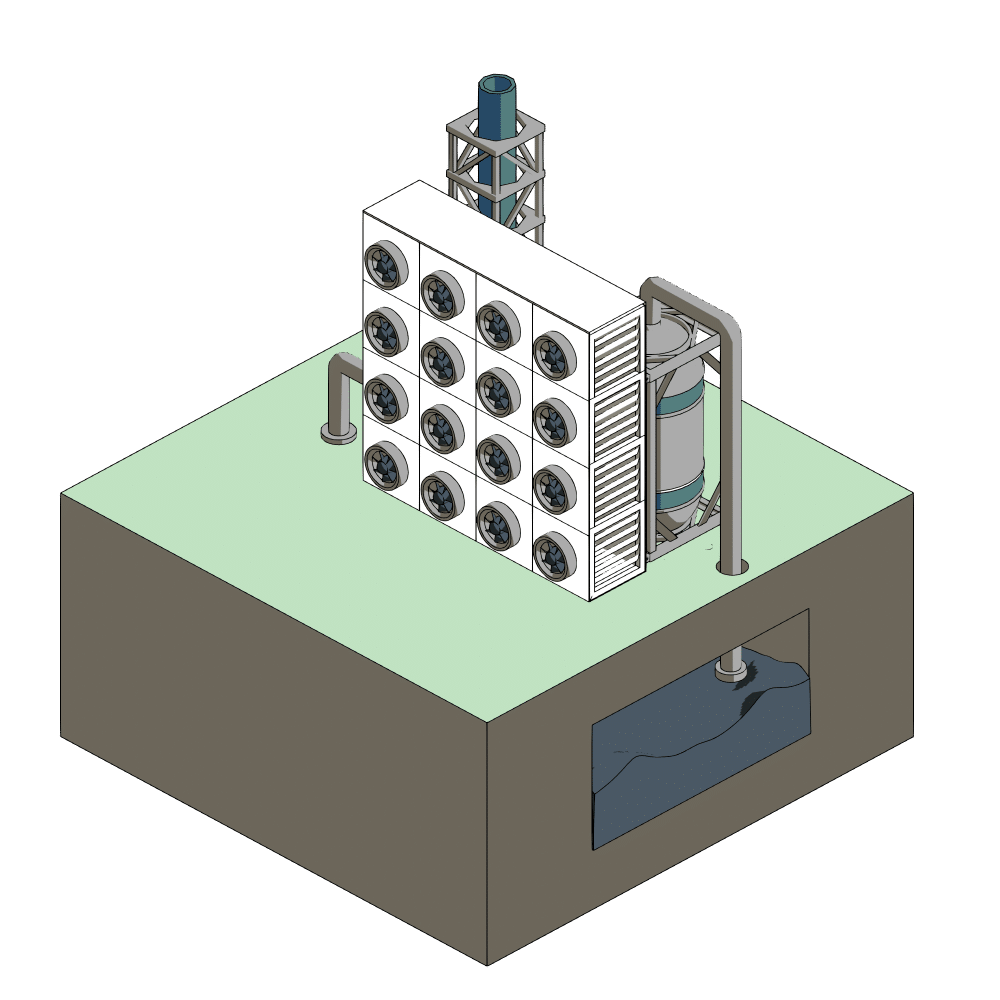
Featured project
Show on map
Latest technology update
Show update
Description and purpose of the technology
DAC is a proposed Carbon Dioxide Removal (CDR) technology that aims to remove carbon dioxide (and potentially other greenhouse gases) from the Earth’s atmosphere directly, on a large scale. DAC projects either aim to inject the captured carbon into underground geological formations for storage, or use it for other purposes such Enhanced Oil Recovery (EOR) and in products of varying durability and longevity.
DAC technologies use chemical substances to selectively react with carbon dioxide in order to scrub it out of the atmosphere. The two most developed processes either dissolve carbon dioxide in liquid solvents such as a strong hydroxide solution, or stick carbon dioxide to the surface of a solid sorbent such as plastic resin.
Several DAC technologies use large fans to move ambient air through filters to enhance the capture process, due to the fact that the carbon dioxide concentration in the atmosphere is in the parts per million range and therefore relatively low. In order to allow the repetitive use of the filters, the captured carbon dioxide is typically released using high temperature (80°C to 800°C), which in turn requires high energy inputs. [1]
Other DAC approaches capture carbon dioxide using battery-like devices, which involve the electrochemical reduction of carbon dioxide or sorbents with humidity-based release processes. The designs of DAC modules vary greatly, and range from shipping containers filled with carbon dioxide collectors to artificial trees. [2]
All forms of DAC are extremely energy-intensive and expensive. The entire capture process for one tonne of carbon dioxide requires between five to 10 GJ of electrical and/or thermal energy. [3] Cost estimates for DAC range from US$ ~100 to ~1,000 per tonne, and claimed lower costs have only been proven theoretically. [4] To have any significant effect on global carbon dioxide concentrations, DAC would need to be rolled-out on a vast scale, raising serious questions about the large amount of energy required and other considerations like the levels of water and land usage required for some DAC technologies, and toxicity impacts from and the disposal of the chemical sorbents used. In addition, safe and long-term carbon dioxide storage cannot be guaranteed by any of the methods currently proposed.
If the captured carbon is to be stored underground through Carbon Capture and Storage (CCS), the captured carbon dioxide must be compressed into liquid form and transported to a site where it can be injected into geological formations. Whilst proponents claim that long-term storage can be guaranteed, the technology comes with a whole range of risks, leakage being the most important one (see Technology Briefing on CCS).
The captured carbon dioxide can also be used in products through Carbon Capture Use and Storage (CCUS), where it is “stored” in goods with varying longevity, such as in soft drinks, carbon-based fuels and building materials. The carbon dioxide usually re-enters the atmosphere quickly when the product is used, so it is at best a postponement of the emissions (see Technology Briefing on CCUS).
The fossil fuel industry is also attracted to DAC because the captured carbon dioxide can be used for Enhanced Oil Recovery (EOR), where it is injected into old oil wells in order to flush out remaining reserves. This is highly carbon-intensive, as some of the carbon dioxide is re-emitted into the atmosphere in the process and more fossil fuels are able to be extracted. A clear example of this is the fact that in 2023 Canadian DAC technology developer Carbon Engineering was bought by Occidental Petroleum for US$ 1.1 billion. Occidental is an international oil and gas company and the largest oil producer in the US Permian Basin.
As with CCS and CCUS technologies, fossil fuel companies and other big polluters are heavily investing in DAC technologies in the hope that they will justify the continued extraction of fossil fuels and avoid the drastic emissions reductions that are urgently required.
Actors involved
Numerous companies and research institutions are commercializing DAC technologies, with millions of dollars being invested into research through private and public sources. Major private investors include fossil fuel, mining and auto manufacturer companies, among them Chevron Technology Ventures, ExxonMobil, the Canadian tar sands mega-investor Murray Edwards, Occidental Petroleum, Shell and Australian oil company BHP. Bill Gates is another major private investor, who also has significant investments in oil transportation. Most public DAC funding has been provided by the USA, the European Union (EU), Canada, Switzerland and Norway. [5]
Many DAC companies are spin-offs from research institutions. [6] Climeworks AG, an ETH Zürich spin-off, currently has more DAC plants than any other company. It commissioned its first plant in 2017 in Hinwil, Switzerland, and is participating in several research projects. According to Climeworks, the Hinwil plant captures around 900 tonnes of carbon dioxide annually and delivers part of it to a nearby greenhouse for carbon dioxide fertilisation. About 600 tonnes are transported by truck to the a Coca-Cola production facility, where sparkling water brand “Valser” is produced. Climeworks also collaborates with several companies to develop and produce synthetic fuels manufactured from captured carbon dioxide, among them the Norwegian company Nordic Blue Crude AS as well as the Italian oil group ENI. Climeworks has received more than € 50 million in public and private grants. [7]
Carbon Engineering Ltd., a company founded by David Keith (lead developer of the Harvard University Solar Geoengineering Research Program and founder of the University of Chicago Climate Systems Engineering Initiative), commissioned a pilot plant in Squamish, Canada in 2015, that captures about a tonne of carbon dioxide a day. In 2017, the plant was connected to a fuel synthesis platform, aiming to produce synthetic transport fuels from captured carbon dioxide and hydrogen. The company has raised more than CAD 100 million from multiple private investors, including oil and mining companies, (Chevron, Occidental Petroleum and BHP) and public sources. It was bought by oil company Occidental Petroleum in 2023, and will develop large-scale DAC plants alongside oil extraction infrastructure in order to use the captured carbon dioxide for EOR. [8]
US-based company Global Thermostat has operated a pilot DAC plant in Menlo Park, California, since 2010. In 2018, it opened its first commercial plant, capturing 4,000 tonnes of carbon dioxide per year, in Huntsville, Alabama. In 2019, the company signed a joint development agreement with ExxonMobil to study the scalability of Global Thermostat’s DAC technology, and as of 2021 has raised more than US$ 70 million in funding. [9]
Other examples of companies commercializing DAC technologies are Finnish company Soletair Power, which has developed a technology that combines DAC, an electrolyser for hydrogen production and a synthesis reactor for hydrocarbon production and commissioned its first demonstration facility in 2018. US-based company Infinitree LLC is developing a carbon dioxide capture system for use in greenhouses, and Dublin-based company Carbon Collect plans to commercialize DAC technology developed at the Arizona State University Centre for Negative Carbon Emissions, involving “planting” 1,200 mechanical trees for carbon dioxide capture. [10]
The world’s largest DAC research program is the EU-funded pan-European research project CarbFix, led by Icelandic company Reykjavik Energy. The project combines DAC with CCS and involves capturing carbon dioxide and hydrogen sulfide at Reykjavik Energy’s Hellisheidi Geothermal Power Plant. Carbon dioxide is dissolved in water under pressure, and the solution is injected into basaltic formations near to the plant, at a depth of 400 to 800 meters, with the aim of mineralizing the gas in the rock. Geothermal Emission Control (GECO) is a follow-up project being conducted to develop the CarbFix process at five demonstration sites throughout Europe. [11]
In the USA, large research initiatives are being developed following the passing of the “Sea Fuel Act of 2019” by congress, instructing the U.S. Department of Defence to implement a research program on DAC. The first phase (2020-2023) involves research and development, followed by testing DAC in demonstration projects (2024-2026). [12]
In Canada, two large research projects began in 2019: The Pacific Institute for Climate Solutions is funding a DACCS (DAC+CCS) project with plans to design a floating platform that would capture carbon dioxide from ambient air and inject it under the seafloor for storage. Natural Resources Canada and industrial partners are also financing a DAC project with the objective of mineralizing carbon dioxide in mine tailings, and trials are being conducted at a nickel mine in British Columbia.
Impacts of the technology
Little information has been published publicly about the capture efficiency and safety of DAC technologies, and critical questions remain over the considerable energy inputs required and the additional greenhouse gas emissions that result, among other issues. For example, the energy required to capture the 600 tonnes of carbon dioxide that Climeworks provides to Coca-Cola each year would be sufficient to supply 760 EU citizens with electricity for a year, and this number excludes other energy-intensive processes such as compressing, purifying and transporting the carbon dioxide over 200 km by truck. [13] With many communities—particularly in the Global South—still without access to electricity, using excessive amounts of renewable energy capacity in this way would be a significant climate injustice.
When deployed on a large-scale, DAC plants require significant energy infrastructure. For a system powered by solar energy, capturing one million tonnes of carbon dioxide annually in a liquid solvent system has been estimated to have a land requirement of 60 to 100 km². This means that rolling-out DAC at a scale sufficient to have an impact on global carbon emissions would be a considerable threat to large areas of natural ecosystems. [14]
A full life-cycle analysis of the construction, maintenance and environmental impacts of large-scale DAC plants has not been made publicly-available, and is a serious knowledge gap. For example, little is known about the toxicity, production and disposal of the carbon dioxide solvents and sorbents that are being used, and the risks of leaks and other sources of contamination. For example, the hydroxide solutions being used, such as Carbon Engineering’s potassium hydroxide solution, require substantial amounts of energy and water during their production, and are highly corrosive. [15]
Water consumption is also an issue for DAC, with one tonne of captured carbon dioxide estimated to require five to 13 tonnes of water, and up to 20 tonnes of water for some solid sorbent-based DAC processes. [16] If scaled-up to climate-relevant dimensions, DAC could exacerbate water scarcity, which is already one of the serious problems being exacerbated by the climate crisis.
The impacts associated with the storage or use of the carbon dioxide captured through DAC processes could also be significant. In the case of geological storage, DAC proponents assume that carbon dioxide can be stored long-term in empty oil and gas reservoirs and deep saline aquifers safely and effectively. However, after decades of research and testing, it appears unlikely that geological storage will ever be able to guarantee reliable long-term storage even at the current scale of CCS, before efforts are made to drastically scale-up injection rates. Mineralization in basaltic rock formations also involves risks, for example in 2018 a group of researchers argued that carbon dioxide injections at the Reykjavik Energy DACCS site Hellisheidi in Iceland induced seismic activity. [17]
When the captured carbon dioxide is used in consumer products, it is usually re-emitted into the atmosphere quickly, and the likely overall result is that more carbon dioxide ends up in the atmosphere than is actually removed. This is due to the large amounts of energy required by DAC processes. Similarly, capturing carbon dioxide to use for EOR results in greater emissions overall.
A modelling exercise looking at the impact of DAC on climate mitigation efforts predicted that it would delay emissions reduction efforts and prolong the use of oil, which would bring positive economic impacts for energy exporting countries. [18] Enabling the continued extraction and use of fossil fuels is one of the main dangers that DAC and other carbon capture schemes pose, a risk that is of course inherent to many geoengineering technologies.
Reality check
DAC technologies have diversified in recent years and there are numerous large-scale trial sites in operation and planned. However, large-scale DAC deployment is only achievable with a major increase in energy production, massive amounts of water and considerable amounts of funding. Whether the captured carbon dioxide can be stored reliably, safely and long-term remains highly uncertain.
Further reading
Geoengineering Monitor (2019), “Direct Air Capture – recent developments and future plans” https://www.geoengineeringmonitor.org/2019/07/direct-air-capture-recent-developments-and-future-plans/
CIEL (2024), Direct Air Capture: Big Oil’s Latest Smokescreen, https://www.ciel.org/wp-content/uploads/2024/04/Direct-Air-Capture-Big-Oils-Latest-Smokescreen-November-2023.pdf
ETC Group and Heinrich Böll Foundation, “Geoengineering Map”, https://map.geoengineeringmonitor.org/
End notes
[1] Beuttler, et al. (2019) The Role of Direct Air Capture in Mitigation of Anthropogenic Greenhouse Gas Emissions, in: Front. Clim., published online: November 21, 2019, https://www.frontiersin.org/articles/10.3389/fclim.2019.00010/full; ETC Group and Heinrich Böll Foundation (2020) Geoengineering Map, https://map.geoengineeringmonitor.org/
[2] ETC Group and Heinrich Böll Foundation (2020)
[3] National Academies of Sciences, Engineering, and Medicine (2019) Negative Emissions Technologies and Reliable Sequestration: A Research Agenda, Washington, DC: The National Academies Press, 510 pages, ISBN 978-0-309-48452-7, https://doi.org/10.17226/25259; Gambhir & Tavoni (2019) Direct Air Carbon Capture and Sequestration: How It Works and How It Could Contribute to Climate-Change Mitigation, in: One Earth, Vol. 1(4): 405-409
[4] Nisbet (2019) THE CARBON REMOVAL DEBATE. Asking Critical Questions About Climate Change Futures, Carbon Removal Briefing No. 2, Institute for Carbon Law Removal and Policy, American University, 24 pages, https://www.american.edu/sis/centers/carbon-removal/upload/carbon-removal-debate.pdf; Fuss, et al. (2018) Negative emissions-Part 2: Costs, potentials and side effects, in: Environmental Research Letters, Vol 13(6): 063002, https://iopscience.iop.org/article/10.1088/1748-9326/aabf9f
[5] ETC Group and Heinrich Böll Foundation (2020)
[6] ETC Group and Heinrich Böll Foundation (2020)
[7] ETC Group and Heinrich Böll Foundation (2020)
[8] ETC Group and Heinrich Böll Foundation (2020)
[9] ETC Group and Heinrich Böll Foundation (2020)
[10] ETC Group and Heinrich Böll Foundation (2020)
[11] ETC Group and Heinrich Böll Foundation (2020)
[12] ETC Group and Heinrich Böll Foundation (2020)
[13] Eurostat (2019) Electricity and heat statistics, accessed: February 2020, https://ec.europa.eu/eurostat/statistics-explained/index.php/Electricity_and_heat_statistics#Consumption_of_electricity_per_capita_in_the_households_sector; ETC Group and Heinrich Böll Foundation (2020)
[14] National Academies of Sciences, Engineering, and Medicine (2019); Nisbet (2019)
[15] Realmonte, et al. (2019) An inter-model assessment of the role of direct air capture in deep mitigation pathways, Natural Communications, Vol. 10, https://www.nature.com/articles/s41467-019-10842-5; National Academies of Sciences, Engineering, and Medicine (2019)
[16] Realmonte, et al. (2019)
[17] Juncu, et al. (2018) Injection-induced surface deformation and seismicity at the Hellisheidi geothermal field, Iceland, Journal of Volcanology and Geothermal Research, Vol. 391, https://www.sciencedirect.com/science/article/pii/S0377027317304080?via%3Dihub
[18] Chen and Tavoni (2013) Direct air capture of CO2 and climate stabilization: A model based assessment, Climatic Change, Vol. 118: 59–72, https://doi.org/10.1007/s10584-013-0714-7
Direct Air Capture
TIPO
Zonas de despliegue
Propuesta
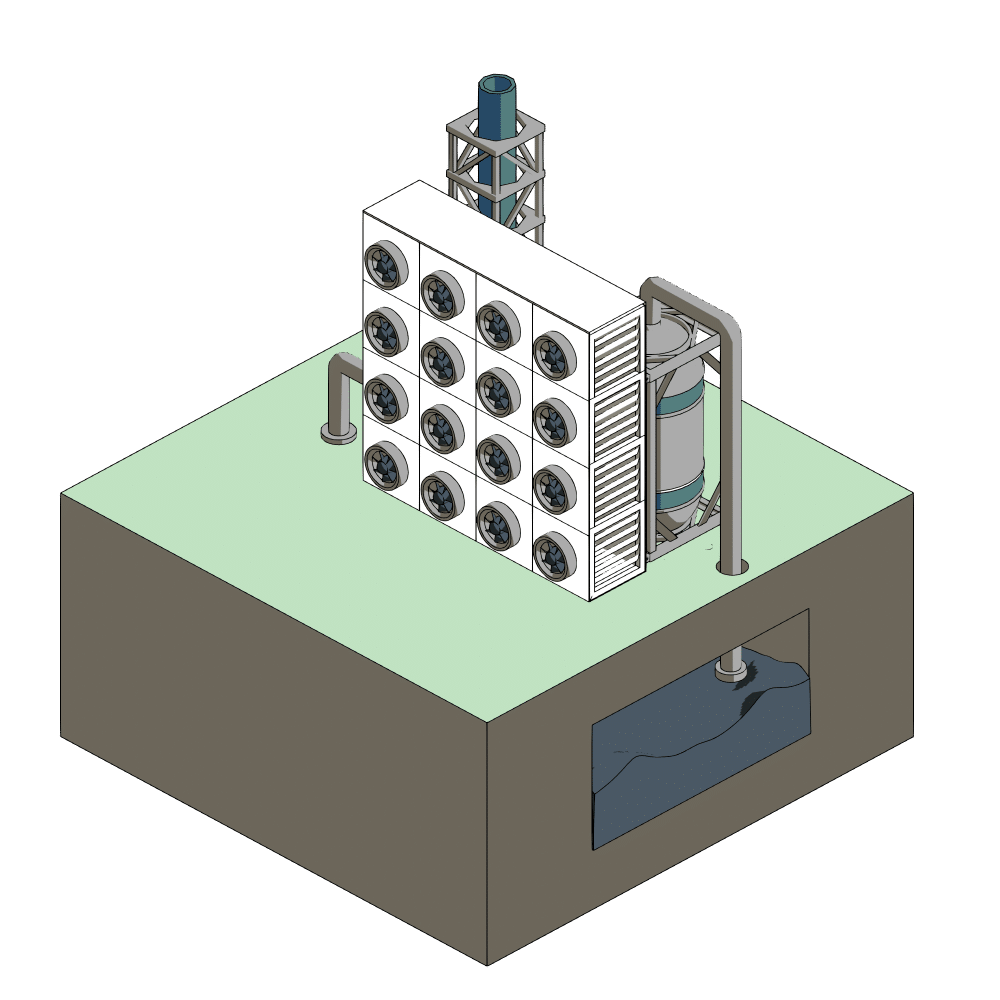
Proyecto destacado
Mostrar en el mapa
Última actualización de la tecnología
Mostrar actualización
Descripción y propósito de la tecnología
DAC is a proposed Carbon Dioxide Removal (CDR) technology that aims to remove carbon dioxide (and potentially other greenhouse gases) from the Earth’s atmosphere directly, on a large scale. DAC projects either aim to inject the captured carbon into underground geological formations for storage, or use it for other purposes such Enhanced Oil Recovery (EOR) and in products of varying durability and longevity.
DAC technologies use chemical substances to selectively react with carbon dioxide in order to scrub it out of the atmosphere. The two most developed processes either dissolve carbon dioxide in liquid solvents such as a strong hydroxide solution, or stick carbon dioxide to the surface of a solid sorbent such as plastic resin.
Several DAC technologies use large fans to move ambient air through filters to enhance the capture process, due to the fact that the carbon dioxide concentration in the atmosphere is in the parts per million range and therefore relatively low. In order to allow the repetitive use of the filters, the captured carbon dioxide is typically released using high temperature (80°C to 800°C), which in turn requires high energy inputs. [1]
Other DAC approaches capture carbon dioxide using battery-like devices, which involve the electrochemical reduction of carbon dioxide or sorbents with humidity-based release processes. The designs of DAC modules vary greatly, and range from shipping containers filled with carbon dioxide collectors to artificial trees. [2]
All forms of DAC are extremely energy-intensive and expensive. The entire capture process for one tonne of carbon dioxide requires between five to 10 GJ of electrical and/or thermal energy. [3] Cost estimates for DAC range from US$ ~100 to ~1,000 per tonne, and claimed lower costs have only been proven theoretically. [4] To have any significant effect on global carbon dioxide concentrations, DAC would need to be rolled-out on a vast scale, raising serious questions about the large amount of energy required and other considerations like the levels of water and land usage required for some DAC technologies, and toxicity impacts from and the disposal of the chemical sorbents used. In addition, safe and long-term carbon dioxide storage cannot be guaranteed by any of the methods currently proposed.
If the captured carbon is to be stored underground through Carbon Capture and Storage (CCS), the captured carbon dioxide must be compressed into liquid form and transported to a site where it can be injected into geological formations. Whilst proponents claim that long-term storage can be guaranteed, the technology comes with a whole range of risks, leakage being the most important one (see Technology Briefing on CCS).
The captured carbon dioxide can also be used in products through Carbon Capture Use and Storage (CCUS), where it is “stored” in goods with varying longevity, such as in soft drinks, carbon-based fuels and building materials. The carbon dioxide usually re-enters the atmosphere quickly when the product is used, so it is at best a postponement of the emissions (see Technology Briefing on CCUS).
The fossil fuel industry is also attracted to DAC because the captured carbon dioxide can be used for Enhanced Oil Recovery (EOR), where it is injected into old oil wells in order to flush out remaining reserves. This is highly carbon-intensive, as some of the carbon dioxide is re-emitted into the atmosphere in the process and more fossil fuels are able to be extracted. A clear example of this is the fact that in 2023 Canadian DAC technology developer Carbon Engineering was bought by Occidental Petroleum for US$ 1.1 billion. Occidental is an international oil and gas company and the largest oil producer in the US Permian Basin.
As with CCS and CCUS technologies, fossil fuel companies and other big polluters are heavily investing in DAC technologies in the hope that they will justify the continued extraction of fossil fuels and avoid the drastic emissions reductions that are urgently required.
Actores involucrados
Numerous companies and research institutions are commercializing DAC technologies, with millions of dollars being invested into research through private and public sources. Major private investors include fossil fuel, mining and auto manufacturer companies, among them Chevron Technology Ventures, ExxonMobil, the Canadian tar sands mega-investor Murray Edwards, Occidental Petroleum, Shell and Australian oil company BHP. Bill Gates is another major private investor, who also has significant investments in oil transportation. Most public DAC funding has been provided by the USA, the European Union (EU), Canada, Switzerland and Norway. [5]
Many DAC companies are spin-offs from research institutions. [6] Climeworks AG, an ETH Zürich spin-off, currently has more DAC plants than any other company. It commissioned its first plant in 2017 in Hinwil, Switzerland, and is participating in several research projects. According to Climeworks, the Hinwil plant captures around 900 tonnes of carbon dioxide annually and delivers part of it to a nearby greenhouse for carbon dioxide fertilisation. About 600 tonnes are transported by truck to the a Coca-Cola production facility, where sparkling water brand “Valser” is produced. Climeworks also collaborates with several companies to develop and produce synthetic fuels manufactured from captured carbon dioxide, among them the Norwegian company Nordic Blue Crude AS as well as the Italian oil group ENI. Climeworks has received more than € 50 million in public and private grants. [7]
Carbon Engineering Ltd., a company founded by David Keith (lead developer of the Harvard University Solar Geoengineering Research Program and founder of the University of Chicago Climate Systems Engineering Initiative), commissioned a pilot plant in Squamish, Canada in 2015, that captures about a tonne of carbon dioxide a day. In 2017, the plant was connected to a fuel synthesis platform, aiming to produce synthetic transport fuels from captured carbon dioxide and hydrogen. The company has raised more than CAD 100 million from multiple private investors, including oil and mining companies, (Chevron, Occidental Petroleum and BHP) and public sources. It was bought by oil company Occidental Petroleum in 2023, and will develop large-scale DAC plants alongside oil extraction infrastructure in order to use the captured carbon dioxide for EOR. [8]
US-based company Global Thermostat has operated a pilot DAC plant in Menlo Park, California, since 2010. In 2018, it opened its first commercial plant, capturing 4,000 tonnes of carbon dioxide per year, in Huntsville, Alabama. In 2019, the company signed a joint development agreement with ExxonMobil to study the scalability of Global Thermostat’s DAC technology, and as of 2021 has raised more than US$ 70 million in funding. [9]
Other examples of companies commercializing DAC technologies are Finnish company Soletair Power, which has developed a technology that combines DAC, an electrolyser for hydrogen production and a synthesis reactor for hydrocarbon production and commissioned its first demonstration facility in 2018. US-based company Infinitree LLC is developing a carbon dioxide capture system for use in greenhouses, and Dublin-based company Carbon Collect plans to commercialize DAC technology developed at the Arizona State University Centre for Negative Carbon Emissions, involving “planting” 1,200 mechanical trees for carbon dioxide capture. [10]
The world’s largest DAC research program is the EU-funded pan-European research project CarbFix, led by Icelandic company Reykjavik Energy. The project combines DAC with CCS and involves capturing carbon dioxide and hydrogen sulfide at Reykjavik Energy’s Hellisheidi Geothermal Power Plant. Carbon dioxide is dissolved in water under pressure, and the solution is injected into basaltic formations near to the plant, at a depth of 400 to 800 meters, with the aim of mineralizing the gas in the rock. Geothermal Emission Control (GECO) is a follow-up project being conducted to develop the CarbFix process at five demonstration sites throughout Europe. [11]
In the USA, large research initiatives are being developed following the passing of the “Sea Fuel Act of 2019” by congress, instructing the U.S. Department of Defence to implement a research program on DAC. The first phase (2020-2023) involves research and development, followed by testing DAC in demonstration projects (2024-2026). [12]
In Canada, two large research projects began in 2019: The Pacific Institute for Climate Solutions is funding a DACCS (DAC+CCS) project with plans to design a floating platform that would capture carbon dioxide from ambient air and inject it under the seafloor for storage. Natural Resources Canada and industrial partners are also financing a DAC project with the objective of mineralizing carbon dioxide in mine tailings, and trials are being conducted at a nickel mine in British Columbia.
Impactos de la tecnología
Little information has been published publicly about the capture efficiency and safety of DAC technologies, and critical questions remain over the considerable energy inputs required and the additional greenhouse gas emissions that result, among other issues. For example, the energy required to capture the 600 tonnes of carbon dioxide that Climeworks provides to Coca-Cola each year would be sufficient to supply 760 EU citizens with electricity for a year, and this number excludes other energy-intensive processes such as compressing, purifying and transporting the carbon dioxide over 200 km by truck. [13] With many communities—particularly in the Global South—still without access to electricity, using excessive amounts of renewable energy capacity in this way would be a significant climate injustice.
When deployed on a large-scale, DAC plants require significant energy infrastructure. For a system powered by solar energy, capturing one million tonnes of carbon dioxide annually in a liquid solvent system has been estimated to have a land requirement of 60 to 100 km². This means that rolling-out DAC at a scale sufficient to have an impact on global carbon emissions would be a considerable threat to large areas of natural ecosystems. [14]
A full life-cycle analysis of the construction, maintenance and environmental impacts of large-scale DAC plants has not been made publicly-available, and is a serious knowledge gap. For example, little is known about the toxicity, production and disposal of the carbon dioxide solvents and sorbents that are being used, and the risks of leaks and other sources of contamination. For example, the hydroxide solutions being used, such as Carbon Engineering’s potassium hydroxide solution, require substantial amounts of energy and water during their production, and are highly corrosive. [15]
Water consumption is also an issue for DAC, with one tonne of captured carbon dioxide estimated to require five to 13 tonnes of water, and up to 20 tonnes of water for some solid sorbent-based DAC processes. [16] If scaled-up to climate-relevant dimensions, DAC could exacerbate water scarcity, which is already one of the serious problems being exacerbated by the climate crisis.
The impacts associated with the storage or use of the carbon dioxide captured through DAC processes could also be significant. In the case of geological storage, DAC proponents assume that carbon dioxide can be stored long-term in empty oil and gas reservoirs and deep saline aquifers safely and effectively. However, after decades of research and testing, it appears unlikely that geological storage will ever be able to guarantee reliable long-term storage even at the current scale of CCS, before efforts are made to drastically scale-up injection rates. Mineralization in basaltic rock formations also involves risks, for example in 2018 a group of researchers argued that carbon dioxide injections at the Reykjavik Energy DACCS site Hellisheidi in Iceland induced seismic activity. [17]
When the captured carbon dioxide is used in consumer products, it is usually re-emitted into the atmosphere quickly, and the likely overall result is that more carbon dioxide ends up in the atmosphere than is actually removed. This is due to the large amounts of energy required by DAC processes. Similarly, capturing carbon dioxide to use for EOR results in greater emissions overall.
A modelling exercise looking at the impact of DAC on climate mitigation efforts predicted that it would delay emissions reduction efforts and prolong the use of oil, which would bring positive economic impacts for energy exporting countries. [18] Enabling the continued extraction and use of fossil fuels is one of the main dangers that DAC and other carbon capture schemes pose, a risk that is of course inherent to many geoengineering technologies.
Visión realista
DAC technologies have diversified in recent years and there are numerous large-scale trial sites in operation and planned. However, large-scale DAC deployment is only achievable with a major increase in energy production, massive amounts of water and considerable amounts of funding. Whether the captured carbon dioxide can be stored reliably, safely and long-term remains highly uncertain.
Lectura complementaria
Geoengineering Monitor (2019), “Direct Air Capture – recent developments and future plans” https://www.geoengineeringmonitor.org/2019/07/direct-air-capture-recent-developments-and-future-plans/
CIEL (2024), Direct Air Capture: Big Oil’s Latest Smokescreen, https://www.ciel.org/wp-content/uploads/2024/04/Direct-Air-Capture-Big-Oils-Latest-Smokescreen-November-2023.pdf
ETC Group and Heinrich Böll Foundation, “Geoengineering Map”, https://map.geoengineeringmonitor.org/
Notas finales
[1] Beuttler, et al. (2019) The Role of Direct Air Capture in Mitigation of Anthropogenic Greenhouse Gas Emissions, in: Front. Clim., published online: November 21, 2019, https://www.frontiersin.org/articles/10.3389/fclim.2019.00010/full; ETC Group and Heinrich Böll Foundation (2020) Geoengineering Map, https://map.geoengineeringmonitor.org/
[2] ETC Group and Heinrich Böll Foundation (2020)
[3] National Academies of Sciences, Engineering, and Medicine (2019) Negative Emissions Technologies and Reliable Sequestration: A Research Agenda, Washington, DC: The National Academies Press, 510 pages, ISBN 978-0-309-48452-7, https://doi.org/10.17226/25259; Gambhir & Tavoni (2019) Direct Air Carbon Capture and Sequestration: How It Works and How It Could Contribute to Climate-Change Mitigation, in: One Earth, Vol. 1(4): 405-409
[4] Nisbet (2019) THE CARBON REMOVAL DEBATE. Asking Critical Questions About Climate Change Futures, Carbon Removal Briefing No. 2, Institute for Carbon Law Removal and Policy, American University, 24 pages, https://www.american.edu/sis/centers/carbon-removal/upload/carbon-removal-debate.pdf; Fuss, et al. (2018) Negative emissions-Part 2: Costs, potentials and side effects, in: Environmental Research Letters, Vol 13(6): 063002, https://iopscience.iop.org/article/10.1088/1748-9326/aabf9f
[5] ETC Group and Heinrich Böll Foundation (2020)
[6] ETC Group and Heinrich Böll Foundation (2020)
[7] ETC Group and Heinrich Böll Foundation (2020)
[8] ETC Group and Heinrich Böll Foundation (2020)
[9] ETC Group and Heinrich Böll Foundation (2020)
[10] ETC Group and Heinrich Böll Foundation (2020)
[11] ETC Group and Heinrich Böll Foundation (2020)
[12] ETC Group and Heinrich Böll Foundation (2020)
[13] Eurostat (2019) Electricity and heat statistics, accessed: February 2020, https://ec.europa.eu/eurostat/statistics-explained/index.php/Electricity_and_heat_statistics#Consumption_of_electricity_per_capita_in_the_households_sector; ETC Group and Heinrich Böll Foundation (2020)
[14] National Academies of Sciences, Engineering, and Medicine (2019); Nisbet (2019)
[15] Realmonte, et al. (2019) An inter-model assessment of the role of direct air capture in deep mitigation pathways, Natural Communications, Vol. 10, https://www.nature.com/articles/s41467-019-10842-5; National Academies of Sciences, Engineering, and Medicine (2019)
[16] Realmonte, et al. (2019)
[17] Juncu, et al. (2018) Injection-induced surface deformation and seismicity at the Hellisheidi geothermal field, Iceland, Journal of Volcanology and Geothermal Research, Vol. 391, https://www.sciencedirect.com/science/article/pii/S0377027317304080?via%3Dihub
[18] Chen and Tavoni (2013) Direct air capture of CO2 and climate stabilization: A model based assessment, Climatic Change, Vol. 118: 59–72, https://doi.org/10.1007/s10584-013-0714-7